Greetings from an undisclosed location in my apartment.
It has been 267 days since the first documented human case of COVID-19.
Housekeeping note:
The in-depth today talks about testing and what we are looking for when we perform various types of COVID-19 tests. It goes deep on a lot of molecular biology concepts, so please reach out via the comment thread or by emailing me directly if you have questions.
I’m going to try and do more in-depth pieces this week since there were none last week while I was on vacation.
Glossary terms are bolded words with links to the running newsletter glossary.
Keep the newsletter growing by sharing it! I love talking about science and explaining important concepts in human health, but I rely on all of you to grow the audience for this:
Now, let’s talk COVID.
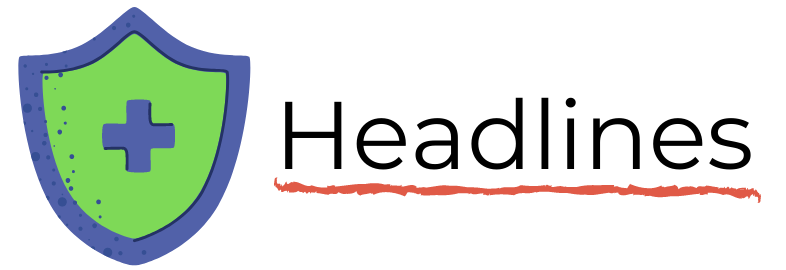
5,000,000 US Cases
Another dark milestone passed this weekend—the US has now reached 5,000,000 today confirmed cases. This is about 5 times the number of Americans who entered 2020 positive for HIV.
Vaccine talk at HHMI
Florian Krammer, an old colleague of mine who has been at the center of coronavirus research in the pandemic—particularly in the development of virus testing and the understanding of how the immune response generates antibodies—did a talk for the Howard Hughes Medical Institute recently that I highly recommend taking a look at:
Florian makes a lot of comments here that I’m going to dissect in an in-depth piece later this week.
Convalescent plasma looks like it works
In April, hospitals in New York started collecting something called convalescent plasma—blood plasma from patients who had recovered from COVID-19 that might contain antibodies against the virus. In other serious virus outbreaks, if antibodies matter to the protective response, convalescent plasma is expected to help fight infection if given to sick people intravenously.
Since we don’t know how well antibodies contribute to protection with SARS-CoV-2, we couldn’t be sure that convalescent plasma would do much. We also weren’t sure if antibodies delivered in the blood would help much with fighting a virus that primarily grows in the respiratory tract and not the bloodstream.
Well, according to a story in Buzzfeed News, it’s looking like convalescent plasma works: https://www.buzzfeednews.com/amphtml/danvergano/convalescent-plasma-eua-fda
This article quotes another former colleague of mine—Dr. Nicole Bouvier—and nicely explains a lot of the issues with validating convalescent plasma as a treatment through randomized, controlled trials. One of the issues, they explain, is that physicians really expect convalescent plasma to work, so they don’t want to enroll patients in a trial of this therapeutic since patients in the trial might get a placebo instead.
There are a number of different data points mentioned in the article, but to me the most interesting is a report from the Mayo Clinic that when patients were given convalescent plasma containing high concentrations of antibodies, they did better (about 10% more survived!) than patients who received plasma with a low concentration of antibodies: https://www.startribune.com/mayo-s-plasma-program-shows-first-signs-of-covid-19-benefit/572021282/
This is what we would call evidence of a “dose-dependent effect”; if you use more of the treatment, you appear to see better outcomes. That’s usually a sign that something works.
If this bears out in further results, it’s also a good sign for vaccine development. We’ve seen that vaccines can yield antibody responses, with those responses sometimes looking better than the antibody levels in convalescent plasma, but we weren’t sure if these antibodies would do anything to protect patients. If convalescent plasma works, though, it supports the idea that antibodies matter for a protective immune response, which bodes well for vaccines.
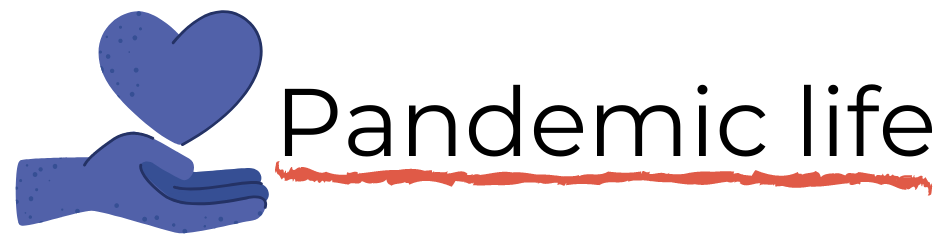
What am I doing to cope with the pandemic? This:
Reading
So close to finishing Jeff Vandermeer’s Acceptance.
Cooking
Yesterday was a whirlwind of cooking because I was trying to kill off some food that came our way when my parents’ house ended up stuck in a power outage. This was more emergency cooking than anything else, so no fancy recipes worth sharing.
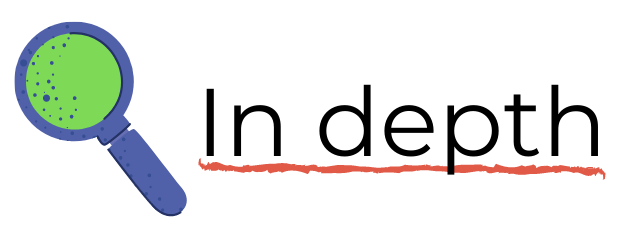
What we detect when we detect SARS-CoV-2
Based on a question from a reader last week, I’d like to discuss the components of the SARS-CoV-2 virus that we can look for as part of a testing technology. If you recall, last week one of the headlines that I discussed was a paper that used “subgenomic RNA” to test for active virus shedding. I received a question about subgenomic RNA, and based on that question I want to walk through some virus components and explain how we can use these to learn about the status of COVID-19 infections in humans.
The complexity of testing for the virus’s presence results from the fact that we can’t readily see the virus, and even under an electron microscope we can’t tell the difference between active and inactive particles. Instead of seeing the virus directly, we have to employ clever techniques to detect molecules that the virus produces, either by copying them in large amounts, or otherwise causing some kind of chemical reaction that helps us to visualize that they are present. To do this, we need to know what parts of the virus to look for.
Let’s start by taking a look at the structure of the virus particle, or virion.
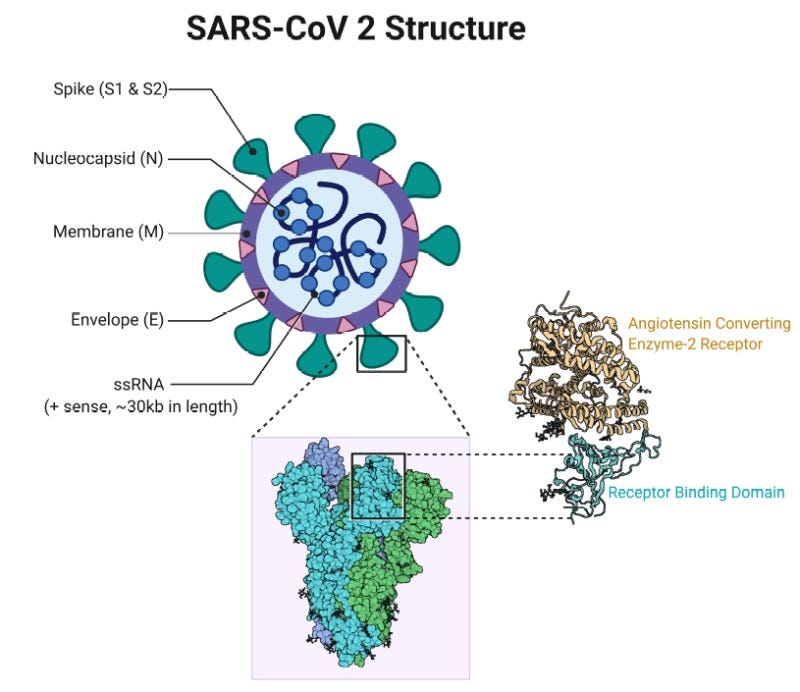
Image is a structure of the SARS-CoV-2 virion, labeling various components that are described in the next paragraph. It also includes a detailed view of the S-protein, showing how it binds to the virus receptor ACE2. Image from: https://www.ncbi.nlm.nih.gov/books/NBK554776/figure/article-52171.image.f3/
The SARS-CoV-2 virion has a limited number of structural components that can identify it; beyond this list there are other components, but they are not something we could test for to definitively identify the virus. The definitive things to look for are: the S1/S2 “spike” glycoprotein, the membrane (M) protein, the envelope (E) protein, the virus genome, and the nucleocapsid (N) protein. Each of these have their own specific function in the virus life cycle, and we will be skipping over those functions for today’s post.
For all of the things labeled “protein,” our testing options are rather limited. These proteins are a source of virus antigens, which will be detectable in bodily fluids of patients who are infected.
Antigen tests
Antigen tests are a classic diagnostic tool in microbiology. If you’ve ever had a strep test in a doctor’s office, the kind where they swab the back of your throat and then wait for a plus sign to appear on a testing strip inside some sort of plastic cassette, you’ve had an antigen test. In that case, the test looks for strep bacterial antigens. It relies on a chemical reaction that depends upon the presence of the antigen. If the antigen is there, the test reacts to its presence and a color change takes place. This is also how pregnancy tests work.
Antigen tests are useful for some specific applications; they are quick, they can be made easily, and they do not take great expertise to administer. Often, antigen tests can be as simple as a paper card that reacts to a sample taken from a potentially infected patient. It would be relatively straightforward to design an antigen-based test that would detect any of the structural SARS-CoV-2 components within a few days of infection.
In fact, there is at least one antigen test already available, which looks for the N protein: https://www.quidel.com/immunoassays/rapid-sars-tests/sofia-sars-antigen-fia
The problem with antigen tests is that while they are quick, they are not very sensitive. They require detectable levels of antigen, and that means that will miss some infections. Other molecular tests are more sensitive and will be more likely to detect infection even at low levels, but they take longer. In a clinical workflow where a rapid antigen test is being used, it is likely that a negative test result would not be considered definitive for a patient who is suspected to be positive either due to symptoms or notable exposure. In the event that such a patient returned a negative antigen test, the clinical team might decide to pursue a more sensitive test anyway.
On the other hand, false positives are not common with antigen tests, so we can be relatively certain that a positive antigen test is an accurate result.
What antigen tests may be good for is screening large groups of people for current infections, and improving the number of people who we are able to isolate quickly. But they can offer a false sense of security because those who test negative may not understand that they aren’t definitively cleared.
Antibody tests
Antigens are also the stimuli that cause the body to produce antibodies. When the immune system produces an antibody, it is reacting to some specific antigen produced by the invader. So, in a way, these antigenic structural proteins that we looked at earlier are also central to the antibody tests that we’ve heard so much about in the news.
The way that antibody tests work is by presenting serum from a patient to a sample of virus proteins, and then watching for a reaction to those virus proteins within the serum sample. There are a few ways that we can visualize that reaction—I won’t belabor these details because there are a number of variations. The general outline of it is that we offer up virus antigen to be bound to by antibodies if they are present in the serum, and then we use some other method to detect if any antibodies bound to the virus antigen presented. This can be done in a laboratory with same-day turnaround.
The thing about antibody tests is that the antibody response is slow. It usually begins to appear within a few days of symptoms appearing, in most virus infections, and increases substantially over a period of 14 to 21 days. This means that we cannot expect to detect antibodies to the virus in a reasonable period following the emergence of symptoms.
What antibody tests can tell us is whether a person has ever been infected with SARS-CoV-2, and that’s something that matters more from a public health standpoint than anything else. Antibody tests have helped us to understand that between 20 and 25 percent of New York City residents were likely infected with SARS-CoV-2 during the first wave of the pandemic.
Antibody tests also have potential uses on an individual level. A negative antibody test can tell you if your isolation procedures have been successful and have kept you free from the virus. This could be useful if you later become infected, as it could help to understand the earliest date on which it is possible for you to have been exposed. I can’t give specific medical advice, but if I were to personally return to working in my office, I would seek out an antibody test. If I tested negative on that, and later became sick with confirmed COVID-19, I would have some reason to suspect the infection was acquired in my workplace and would let my employer know about that. It’s possible that this could induce my employer to take some responsibility for costs associated with treating my hypothetical infection. I can’t speak to whether or not that would actually happen, but it’s a potential application of antibody tests on an individual level.
RT-PCR and genome products
The other way to detect virus infection is to look for the RNA molecules that are made by the virus. We can detect these directly by using a process called RT-PCR. RT-PCR stands for reverse transcriptase-polymerase chain reaction. Reverse transcriptase is an enzyme, derived from viruses in the same general lineage as HIV, that turns RNA into DNA. PCR is a tried and true technique that exponentially copies of specific DNA, doubling it with every cycle of replication, a process that is called “amplification”. The power of doubling means that RT-PCR can be very sensitive to the presence of virus RNA; you might have noticed news stories about people who still test positive for virus RNA for months after they have gotten better from COVID-19. This is because of the sensitivity of RT-PCR.
This video explains how RT-PCR works more visually:
When we do RT-PCR, most of the time we are looking for the virus’s RNA genome, but it’s only one of many RNA products that are made from coronaviruses.
In your cells, RNA is a bit of a workhorse. It communicates messages from your genome to create to proteins. It is incorporated into structures that are able to execute various cell functions. It sends additional signals that regulate your genes.
In an RNA virus, the genome itself is RNA. Specifically, coronaviruses are what’s known as a single-stranded, positive-sense, nonsegmented RNA virus. This means that their RNA genome is not a double helix like our DNA genome; there is just one strand, and it does not necessarily fold into a helix. Positive-sense means that the genome itself is in the appropriate formation to be translated into protein without a second strand first being transcribed. Nonsegmented means that the genome is a single piece; this is different from our own genome, which is in 23 pieces known as chromosomes, or the influenza virus genome, which is separated across 8 segments.
The upshot of all this is that the virus can begin executing instructions to create proteins directly from its genome within a short time after entering the cell. There’s a lot more to the coronavirus genome than that, though. Strap in, because things are about to get weird.
The genome of a coronavirus doesn’t directly code for everything that the virus needs to replicate. For one thing, the virus needs to copy its genome in order to replicate. This means that it has to make the complementary strand of its genome—the “negative” sense strand that we take for granted with our double-stranded, helical DNA genome. This negative sense template for making more genome copies is called, creatively, the “antigenome.”
The antigenome is a template for creating more copies of the genome, but it’s also much more than that. Viruses, and RNA viruses in particular, have ridiculously small genomes. A human has about 3,000,000,000 individual letters in the genome. A coronavirus has 30,000—and that’s a lot for an RNA virus. To make up for this lack of room, viruses have a lot of creative ways of packing additional genes into their genomes. One way that coronaviruses do this is by a process known as “discontinuous transcription.”
Discontinuous transcription leads to the creation of subgenomic RNAs. During discontinuous transcription, the virus RNA polymerase creates RNAs that are of the opposite sense of whatever molecule it’s starting from, and then doesn’t fully transcribe it. It creates something smaller, a subgenomic RNA. What is thought to happen is that the virus polymerase creates a negative-sense subgenomic RNA from the full genome, and then this is copied again into a positive-sense subgenomic RNA that can be translated into proteins. Yes, it’s weird and complicated, and frankly since I’m not a coronavirus expert I’m sure there are some subtleties that I’m missing here. But, what’s important is that this mechanism allows the virus to pack a lot of different messages into a small genome and gives us several different types of RNA that we can detect.
So to review, there are four general types of RNA that are made in a coronavirus infection:
The positive-sense genome
The negative-sense antigenome
Positive-sense subgenomic RNA
Negative-sense subgenomic RNA
These molecules contribute to the complex ballet that causes the virus to replicate itself, package itself into new virions, and get back out of the cell, but only one of them—the genome—is efficiently packaged into virions. Let’s get back to testing, though. RT-PCR can be very specific. We can detect the whole genome, or we can detect subgenomic sequences. It is a tool that allows us to detect whatever specific sequences we want to detect because we choose the sites that we amplify. So we can create sets of “primers” that define the amplified sequence to be whatever we want them to be.
This means we can specifically detect the genome, or we can specifically detect the subgenomic RNAs.
As a test, RT-PCR is sensitive, but it is not specific for virus infection, at least not in its current incarnation. Like I mentioned before, genomic RNA can be detected in patients long after they have cleared their virus infection. The most common version of the RT-PCR test for virus looks for the presence of virus genome copies. Copies of the RNA genome can persist within the body for a long time after infection resolves, and this can make for misleading test results. These genome copies aren’t necessarily infectious virus; they don’t mean that a person is still actively infected or that they are producing virions.
In the paper we talked about last week, the authors tried to get around this by creating an RT-PCR primer set that is specific to the subgenomic RNAs. Because these RNAs do not go into virions to the same degree that virus genomes do, the authors figured that the subgenomic RNAs would only be detectable when the virus infection is active in a patient.
They then went ahead and showed that you could only recover active virions from patients for about 7–8 days after symptom onset, and that you also could only detect subgenomic RNAs by RT-PCR in this same window. This is a really interesting breakthrough because it suggests a way that we can develop an RT-PCR test that is better at telling us whether someone has an active infection or not.
I wonder if this approach will be used by any of the companies working on COVID tests to make more accurate diagnostics that give us a better sense of whether or not someone is actively infected. If it does get used, it will be important that such a test return results quickly, something that we’ve had problems with here in the US. It’s not clear how useful it would be to find out you had an active infection three weeks ago, when we think that infections only last about that long in the first place. We’ll just have to keep an eye on the testing landscape and see how it develops.
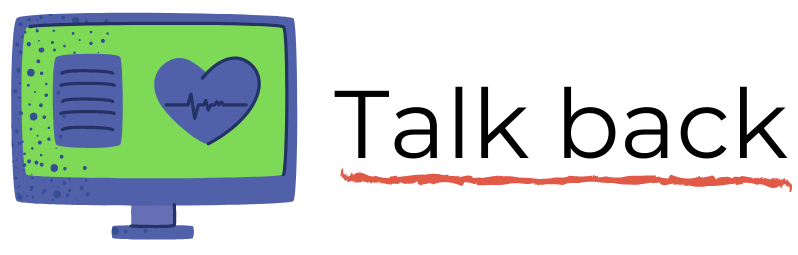
Join the conversation, and what you say will impact what I talk about in the next issue.
I know there was a lot of technical stuff today, so please jump in to the conversation and ask questions, too.
Also, I welcome any feedback on structure and content. I want this to be as useful as possible, and I can only make that happen with constructive comments.
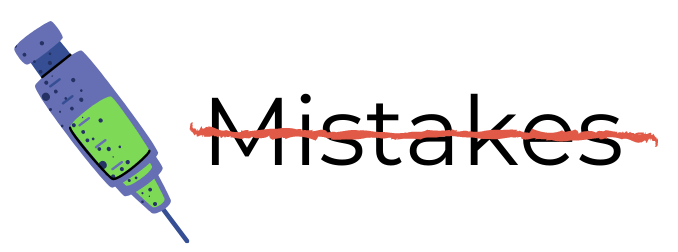
This newsletter will contain mistakes. When you find them, tell me about them so that I can fix them. I would rather this newsletter be correct than protect my ego.
Though I can’t correct the emailed version after it has been sent, I do update the online post of the newsletter every time a mistake is brought to my attention.
No corrections since last issue.
See you all next time. Have a good week, everyone!
Always,
JS