Greetings from an undisclosed location in my apartment.
It has been 277 days since the first documented human case of COVID-19. One of these days I am going to forget to update this and you’re all going to be worried you’re stuck in a time loop. If this happens, rest assured it’s probably just my fault.
Housekeeping note:
Short headlines today because there’s an in-depth and because I am told there is some news in politics, which seems to be reducing COVID-19 coverage a little.
The in-depth today is the start of a two-part series that will cover reasons that we know that SARS-CoV-2 is not of manmade origin.
Glossary terms are bolded words with links to the running newsletter glossary.
Keep the newsletter growing by sharing it! I love talking about science and explaining important concepts in human health, but I rely on all of you to grow the audience for this:
Now, let’s talk COVID.
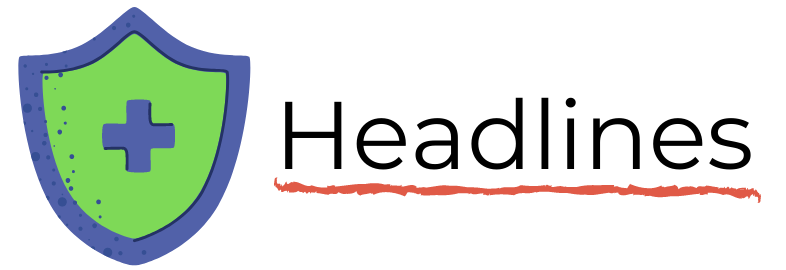
The first limited evidence that SARS-CoV-2 neutralizing antibodies correlate with protection
The New York Times reports on a story in which three sailors on a fishing boat were unaffected by an outbreak of COVID-19 that occurred on that boat: https://www.nytimes.com/2020/08/19/health/coronavirus-fishing-boat.html
Of 122 crew, 103 became infected. All had been tested before departure for both virus and antibodies, and among the 19 who did not become infected, 3 had tested positive for antibodies before departure. They were the only 3 sailors who were positive for SARS-CoV-2 antibodies at the outset of the voyage.
This does not prove that antibodies equal protection, and the study is neither peer reviewed nor large enough to demonstrate that antibodies are a true correlate of protection. But this is the first evidence that we have of a protective effect associated with having antibodies, and at present, it’s pretty much our best evidence of that.
The New York Times article covers some other aspects of this also and is well worth reading.
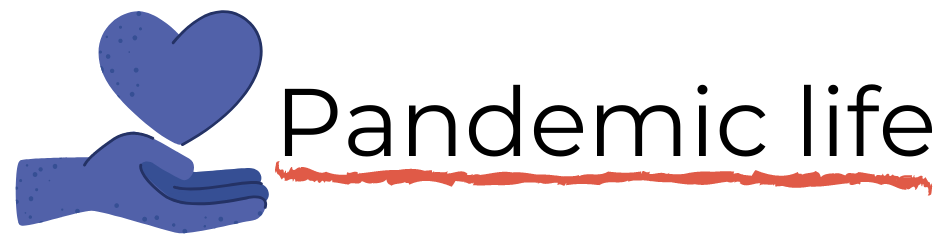
What am I doing to cope with the pandemic? This:
Reading
I’ll be starting the next book in Ursula K LeGuin’s Chronicles of the Western Shore series. I’ll let you know how it goes. The last book in the series is what I’m really hoping to get to, since it won a Nebula Award, but I like to read things in context.
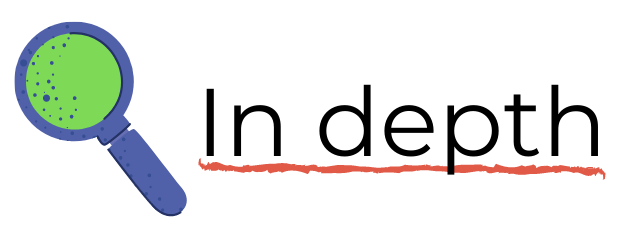
How do we know it wasn’t made in a lab? (Part 1)
Early in the COVID-19 pandemic there were numerous people around the world who theorized that the virus might have originated in a laboratory. They were wrong, and we knew they were wrong from before they began to speculate. I would like to explore why.
I want to set the stage with something from my personal experience. You should not consider this “data,” per se, because it is an anecdote, but it is a meaningful one. The story is this: at one point in my life, I had all the knowledge and materials necessary to design and grow viruses to my own specifications. In fact, I did grow viruses to my own specifications.
In molecular biology, “clone” has a very specific meaning. A clone is a copy of a specific genetic sequence, used for laboratory experimentation or other purposes. A molecular clone of a virus, in many cases, can be used to create copies of that virus for laboratory work, or even for applications like creating influenza vaccines. When I was in the lab, I was able to design infectious clones of viruses like influenza A, the measles virus, and Newcastle disease virus (the latter being a poultry pathogen), put those clones into cells, and produce infectious viruses that could infect me, you, or—importantly—the cells I was working with to study the viruses.
What’s really powerful about this technique is that biologists have a large repertoire of tools at our disposal that we can use to edit genetic material. It’s not even very difficult to do. It takes a day or two, maybe, to edit a specific sequence in the way that you want, if you’re clever about how you design your strategy for the editing.
This means that I could not only clone the virus as it is found in the wild—the “wild type”—but also introduce my own changes that would serve my experimental purposes. Make the virus contain a glowing protein that lets me see what cells it has infected? Fine! Add one protein from a dangerous virus to a virus that can’t replicate in human cells, so I can safely study the one protein? Did that. Create a chimeric influenza virus that carries antigens from two different influenza strains? Did it, got the t-shirt. Specifically, the t-shirt was a giveaway from a company that sells kits for measuring cell death.
Anyway, I’m saying all of this to establish that not only have I worked with viruses before, I have created them from scratch using genetic material only, and I have edited them to my own specifications. These are the skills that would be required to create a novel coronavirus in a lab.
What I have left out of this is something extremely important, though: it’s really hard to do this! I can’t even totally explain how hard it is in a single article while still having time to walk through why SARS-CoV-2 isn’t an engineered virus, which is why this is going to be two pieces.
The broadest answer to why it’s so difficult is that viruses are optimized in the laboratory of nature. In a single drop of lung fluid from a flu infection, there might be a million influenza virus particles. In your lungs in total? It’s thousands of times that. Then consider the fact that tens of millions, if not hundreds of millions, of people get infected with influenza viruses each year. A ludicrously large number of influenza virus particles are made in human lungs each year. And influenza has been around longer than that.
The total length of the influenza virus genome is 10,000 RNA bases, give or take. By good experimental evidence, we know that there is at least one meaningful mutation in every 10,000 RNA bases copied by influenza virus RNA-dependent RNA polymerase. This means that if you can find 10,000 influenza virus particles each with different mutations, you’ve gotten coverage of the entire genome. As we’ve established, each year many many millions of times that many influenza viruses, each with at least one mutation, are created.
To replicate this in a lab through intentional mutation, you would need hundreds of millions, if not billions, of graduate students working to create each of these viruses. While some professors may dream of such a scenario, most realize that it’s not an option. The fact is, no matter what we may try to do to edit the influenza virus, nature has already done the experiment and the existence of the virus that we are trying to edit is proof that the experiment doesn’t create a virus that works very well.
Coronaviruses are different from influenza viruses. They contain about 30,000 RNA bases and they mutate less frequently than influenza viruses, but at the scales at which viruses are produced, these are rather minor facts. By pure chance, coronaviruses have also explored what I like to think of as their “mutational space” and found a balance of genetic elements that make them work to infect and spread. It would be quite difficult for humans to do better than nature has done; any change we make is likely to come at an extreme cost to the virus. My laboratory notebooks from when I was a bench scientist contain a large amount of proof that most things you try to change totally ruin the virus you’re working with and make it impossible to accomplish anything.
Part of the reason is that viruses exist under tremendous constraint. The cells that they infect are on constant lookout for infections, and in order to survive, viruses must avoid detection so they are not destroyed. Viruses must have functions that allow them to replicate via host machinery, and they must remain compatible with that machinery because all the tools that are needed to replicate the virus would never fit within the virus particle itself. In fact, that’s definitional—if all the tools fit, it wouldn’t be a virus. It would be a cell, and it wouldn’t need to infect other cells in order to copy itself.
Speaking of fitting things, viruses are often evolved to optimize the space within their outer bounds. Packaging a genome, a small number of important accessory proteins, etc. within a coating of protein or fat is not trivial. Entire careers focus on how specific viruses have evolved to accomplish packaging. Part of that is making sure that the parts of the virus are compatible with each other, and compatible with host machinery that takes them to where they are put into the new virus particle. Part of it is just making sure they still fit right into the new particle, because there’s only so much room in the proverbial suitcase.
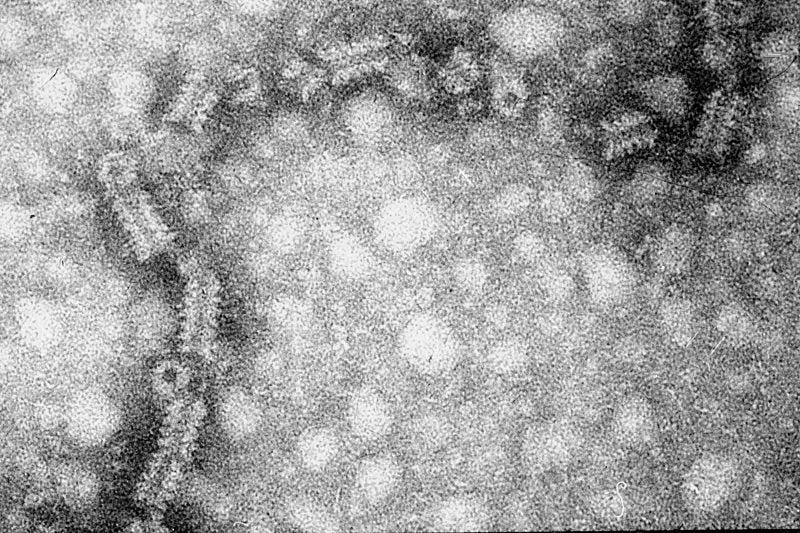
Image is an electron microscope picture, in black and white, of measles virus nucleoprotein, which adopts a helix-like structure. Image from Dr. Graham Beards, on wikimedia commons: https://commons.wikimedia.org/wiki/File:Mumps_virus_electron_micrograph.jpg
An example of this can be found in how paramyxoviruses, like the measles virus, have evolved a means of protecting their genome molecules. A protein called the nucleoprotein binds tightly to the paramyxovirus genome, and this helps to protect the genome from cellular responses that could destroy it.
There’s something else interesting about paramyxoviruses—they must always have a genome length that is divisible by six, although their genomes are around 12,000 to 20,000 nucleotide bases in length. Weird, right? Divisible by seven, or 5—won’t do. It has to be six. As it turns out, this is because of the nucleoprotein. Individual nucleoprotein molecules bind to six nucleotide bases in the genome. If it’s not divisible by six, the genome won’t be bound properly by nucleoprotein and won’t be protected or functional. This obviously puts constraints on what a “survivable” mutation is for a paramyxovirus; if it affects divisibility by six, it won’t work. If it affects the number of nucleotides that the nucleoprotein can bind, the virus also won’t work. There is a tightrope to walk.
This sort of feature of paramyxoviruses is not unusual among other species, though this example is a particularly stark one. Yet for all other viruses, the parts have to fit together right so that the virus can get in to the cell, copy itself, get out, transmit, and survive, and they all have to do that with a careful balance that leads to dozens of individual virus features like the “rule of six,” or like the delicate process of packaging 8 individual influenza virus genome segments into a single virus particle, or like many other examples. Each of these processes is not very tolerant to mutation.
I hope that this illustrates the engineering constraints that viruses operate within. If we understand these constraints, then it becomes clear how easy it is to break a virus. A single mutation can throw off some delicate balance, and large numbers of mutations are even more likely to cause problems.
To engineer a virus like SARS-CoV-2 from its ancestral bat viruses, in the clumsy human laboratories of the world, would require a large number of mutations. The virus sequence combines information from virus lineages that are most often found separately. It is not obvious at all that these pieces of information would be compatible with one another, and to weld them together under laboratory conditions from amongst the tremendous number of options that exist would, to my mind, take longer than the 5 decades in which people have been able to engage in molecular cloning. Certainly it would take longer than the 17 years since we first discovered the SARS coronaviruses.
This establishes that it would be extremely difficult, if not impossible, to create a virus of this type in the lab, but that is an argument is incomplete. I do not only want to provide reasons that this is unlikely to have come from a lab; I also want to demonstrate reasons that it is likely to have come from nature.
In the next installment of this series, I will address specific evidence for both parts of this logical construct, as it applies to SARS-CoV-2, walking through the precise, documented reasons that it is clear that the virus was not made it in a lab, and also the precise, documented reasons that it is clear that the virus did originate in nature.
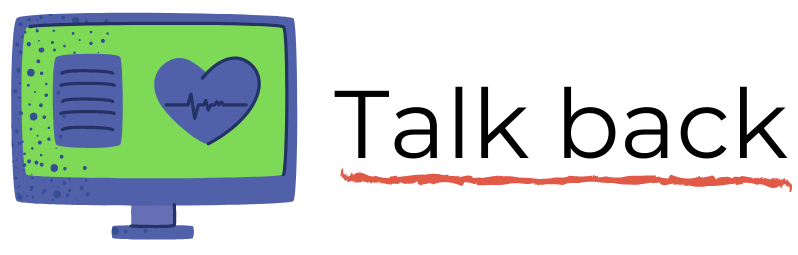
Join the conversation, and what you say will impact what I talk about in the next issue.
Also, let me know any other thoughts you might have about the newsletter. I’d like to make sure you’re getting what you want out of this.
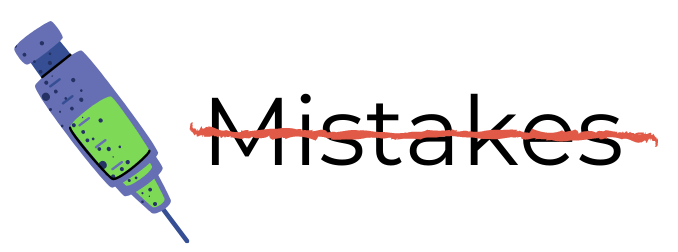
This newsletter will contain mistakes. When you find them, tell me about them so that I can fix them. I would rather this newsletter be correct than protect my ego.
Though I can’t correct the emailed version after it has been sent, I do update the online post of the newsletter every time a mistake is brought to my attention.
No corrections since last issue.
See you all next time.
Always,
JS